Virtually all the potable water consumed in the Centre Region is drawn from the groundwater reservoir. Even residents in the Bellefonte area depend on groundwater that emerges naturally in the Big Spring. But the movement of water on and beneath the surface is controlled by the rocks and structures that shape the valley.
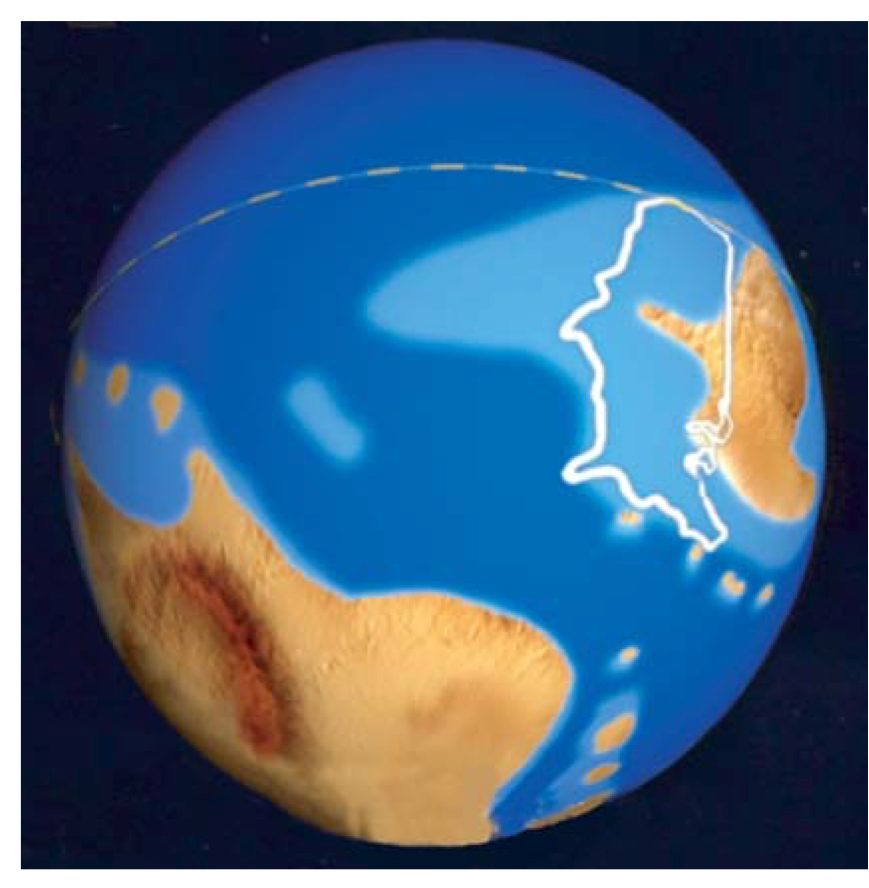
Figure 1. Paleogeographic globe with present-day North America superimposed on the American landmass of c. 450 Ma. (White dashed line is the equator; north is at the top.) (U.S. Geological Survey)
About 500 million years ago the land that is now North America was part of a large landmass that included much of Arctic Canada, Alaska, and Greenland. The land we call central Pennsylvania lay beneath the water of a shallow sea, much like the modern east coast continental shelf but located near latitude 30° South (Figure 1).
Although the land was devoid of life, this was a time (Cambrian period) of an explosive growth of life in the sea (See Appendix 1 for geologic timeline). That growth is marked by an abundance of ‘hard parts’ preserved as fossils in the rocks. One group of animals developed jointed exoskeletons of chitin (like modern insects), others built individual shells or communal structures of calcium carbonate (like modern clams and corals). Marine rocks deposited after this time often contain such fossils, and in waters remote from land, biological material, especially calcium carbonate shell debris, may be the dominant sediment.
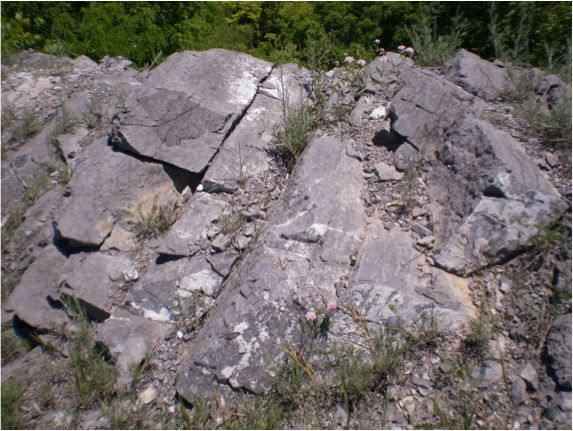
Photo 1. Trenton limestone, deposited as a fine carbonate mud in the shallow, early Paleozoic sea. (Oak Hall Quarry) Photo by R. Schmalz
The oldest rocks exposed in the Nittany Valley date from this period of earth history. They are carbonate rocks deposited in that shallow sea far from most sources of terrestrial sediment (Photo 1). Several large islands along the eastern margin of the shelf contributed very little terrestrial sediment, although volcanic islands added a few thin beds of wind-blown ash. The eastern margin of this ancient ‘continent’ may have resembled the present-day margin of Asia, the Sea of Japan and the Japanese islands.
Abundant ripple marks, mud cracks and other evidence show that the water covering the shelf was shallow and periodically exposed to the air. The earliest carbonate sediments deposited on the shelf are composed mainly of dolomite, a carbonate mineral containing both calcium and magnesium. This suggests that the water covering the shelf was not only shallow, but very salty as well. This would correspond with its position in what today is the desert climate zone (25 - 40 ° north and south latitudes).
To the north and west, the shelf sea was bounded by a large landmass from which sand sometimes washed into the sea. As a result, some of the dolomite beds are sandy, and there are a few interlaminated beds of relatively pure sandstone. Because of their porosity, these sandy beds play a critical role in the movement of groundwater in the Centre Region today.
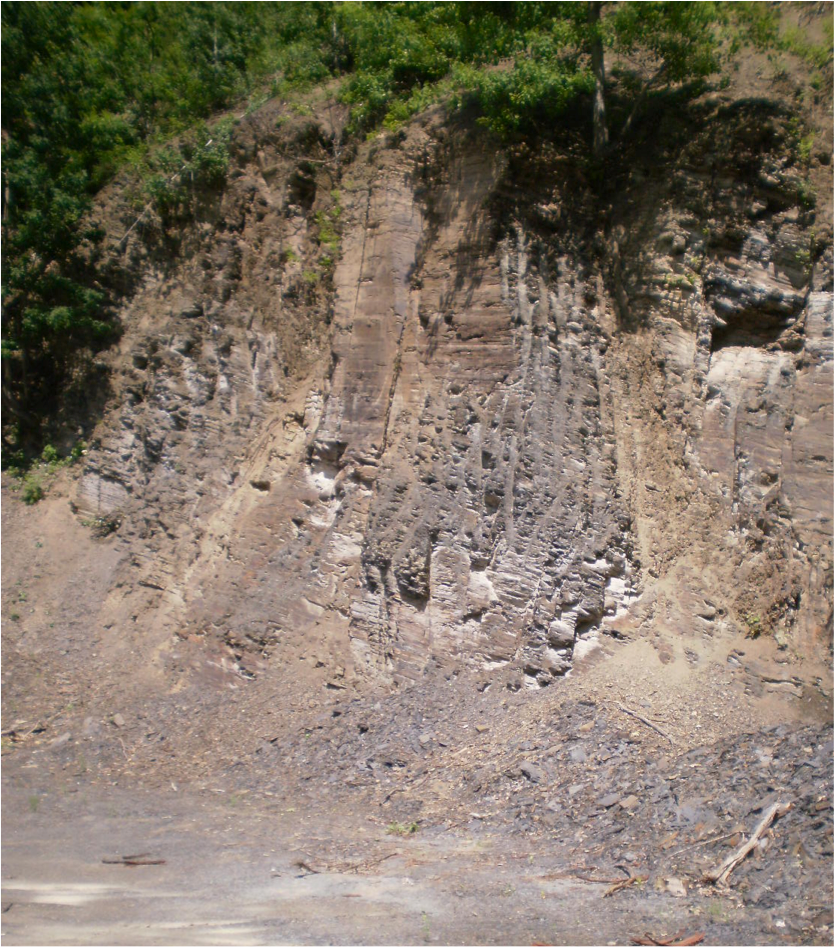
Photo 2. Reedsville shale in the shale pit just outside of Pleasant Gap. Photo by R. Schmalz.
During the next two hundred million years, tectonic forces carried a second large landmass that included modern Africa slowly but inexorably westward. This movement gradually closed the ancient eastern ocean, and eventually led to a collision between the two landmasses. In its earliest phase, the movement thrust the offshore islands against and eventually onto the continental shelf, forming an ancient (and now long gone) Acadian Mountain range. The increased weight caused the continental margin to subside, converting the shelf sea into a basin in which dark colored mud was deposited (the (Ordovician) Reedsville shale, Photo 2). As the basin filled, the sediment became coarser and the black shale was replaced by dark greenish-gray sandstone that grades upward into reddish-gray (locally almost pink) sandstone (the (Ordovician) Bald Eagle sandstone, Photo 3). The sandstone is overlain in turn by a thick series of inter-bedded red and gray mud, silt and sand deposits of a tropical coastal plain (the (Ordovician) Juniata redbeds).
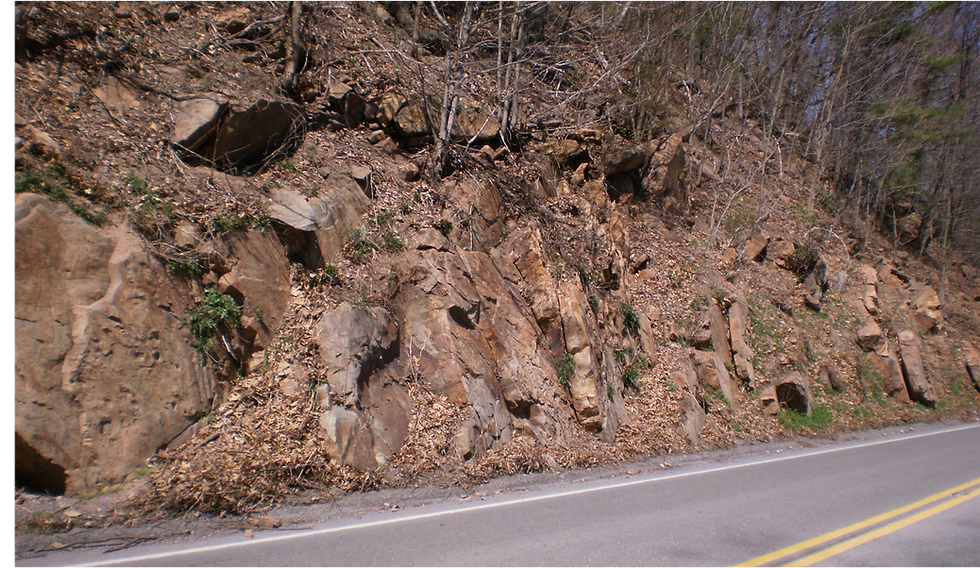
Photo 3. Oswego or Bald Eagle sandstone at the crest of Nittany Mountain on State Route 144. Photo by R. Schmalz.
Finally, when the Acadian Mountains had been largely reduced by weathering and erosion, and perhaps aided by a minor rise in sea level, the weathered debris was reworked and winnowed to build a vast beach deposit or offshore sand bar of nearly pure quartz sand (the (Silurian) Tuscarora sandstone).
The Tuscarora sandstone (or quartzite), deposited about 430 million years ago, is the youngest rock still exposed in the Nittany Valley. But it was originally overlain by approximately 8,100 feet of additional sedimentary rock, ranging in age from Silurian through late Carboniferous. These rocks were largely of continental origin and included marsh deposits that are now coal beds. The younger rocks preserved in the Allegheny Plateau have been completely removed by erosion in the area of the Nittany Valley.
By 250 million years ago, a total of nearly 21,000 feet of sedimentary rocks had been deposited in the Appalachian Basin. When the basal (Cambrian) carbonate rocks were deposited, Pennsylvania lay near the margin of the temperate climate zone of the southern hemisphere. By the end of the Permian period (250 million years ago), the American landmass had drifted northward through the southern desert climate zone and tropics to the equator. This slow northward drift continues to the present day.
About 250 million years ago, the westward drift of the African landmass culminated in a collision with the American landmass near modern Philadelphia. This was no instantaneous event like a modern ‘fender-bender’, but a prolonged process lasting millions of years. The entire sequence of sedimentary rocks in the Appalachian Basin was crushed and uplifted to form a mountain range of alpine proportion extending from Alabama to Maine (and beyond).
In the area of present-day Philadelphia, frictional heat generated by the impact heated some rocks almost to the melting point (the Wissahickon schist). Inland, the collision thrust great slabs of rock upon one another and crumpled the rocks into a series of folds. The intensity of deformation diminished to the northwest, and major folding ended at what we call the Allegheny Front. Subsequent weathering and erosion reduced the mountains to an almost flat plain upon which only the most resistant rocks, the ‘bare bones’ remain standing.
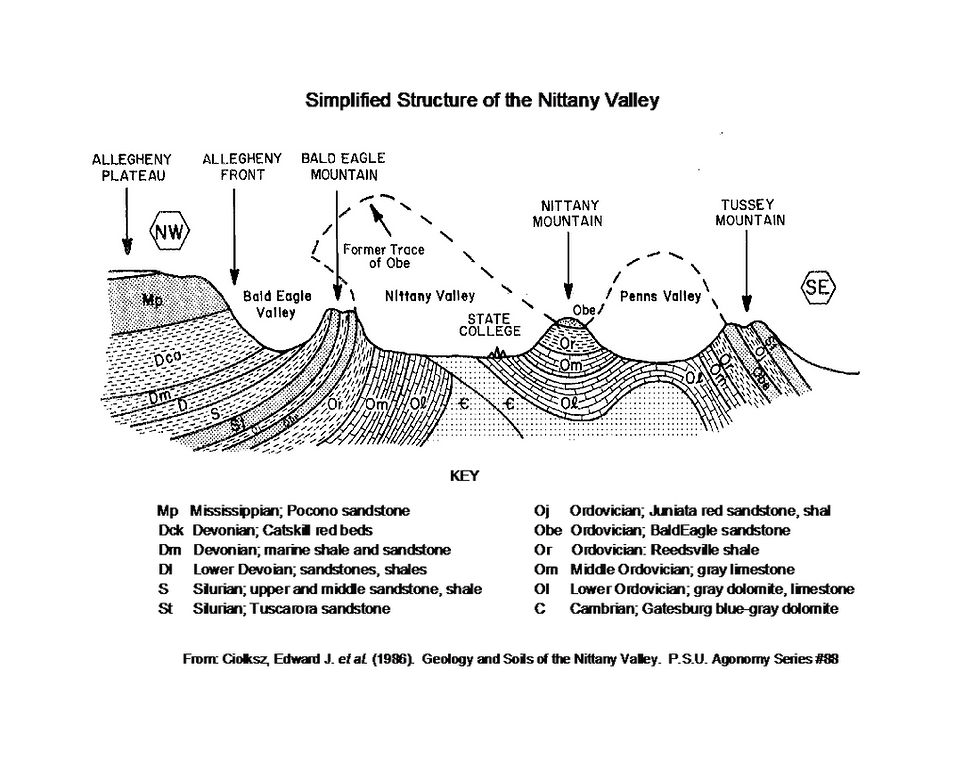
Figure 2. Simplified cross section of the Nittany Valley (P.S.U. Agronomy Paper, #8)
But geologists have reconstructed the ancient Appalachian Mountains based on present day topography and the attitudes of exposed rock outcrops. Near the northwestern margin of the fold belt, the rocks were raised in a broad arch that extended from Tussey Mountain to Bald Eagle Mountain over the Nittany Valley. To the northwest, the Tuscarora sandstone is overlain by younger rocks in the Allegheny Plateau (Figure 2). The oldest rocks involved, the dolomites and limestones of the ancient shelf sea are exposed in the Valley floor (Photo 4). The bounding ridges have double crests supported by the resistant Bald Eagle and Tuscarora sandstones, separated by a shallow swale underlain by the Juniata redbeds. The lower slopes of both mountains are composed of dark Reedsville shale.
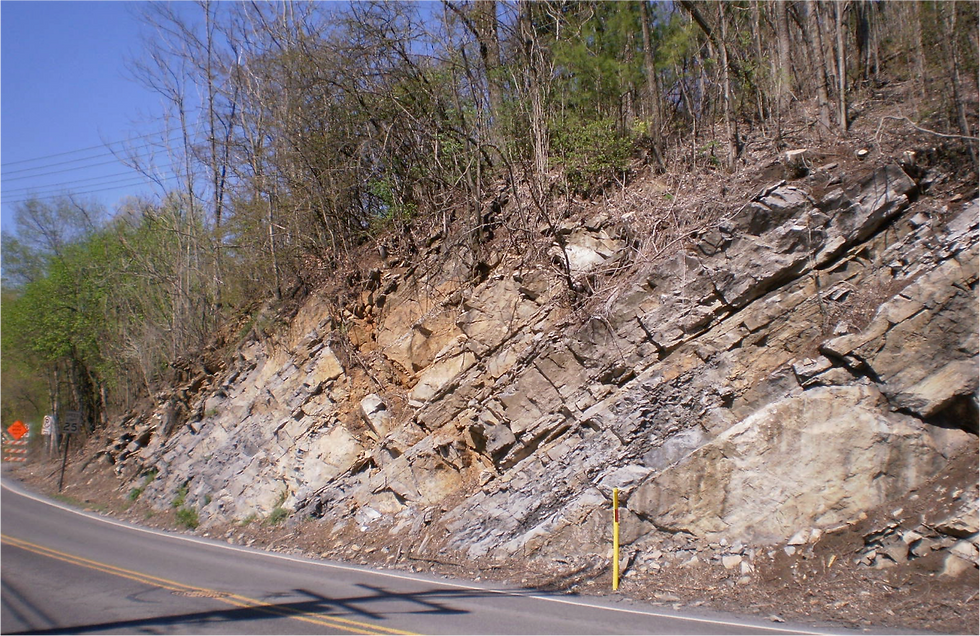
Photo 4. Lower Ordovician carbonate rocks along State Route 144 near Axemann. Photo by R. Schmalz.
In large measure, these rocky remnants determine the movement of surface water. In central Pennsylvania (the “Valley and Ridge Province”), streams and rivers are trapped between resistant rock ridges like Tussey and Bald Eagle Mountains. Their general northeast-southwest flow is parallel to the Atlantic coast rather than southeastward toward the sea. Fractures or other weaknesses in the rock of the ridges afford passages for the escape of surface streams like the Susquehanna and Delaware rivers, and are widened by erosion to form water gaps like that at Milesburg. The result is a regional drainage pattern that resembles a garden trellis with streams flowing alternately parallel to and at right angles to the ridges. The movement of groundwater, described elsewhere, conforms to regional geology in much the same way.
(Demographers point out that the human development of the region has been shaped by the same constraints. Railroads and highways followed the same trellis-like pattern, with urban centers at critical junctions.)
Robert Schmalz was Professor of Geology at Penn State University, and taught several undergraduate courses. He was mainly interested in the chemistry of natural waters.
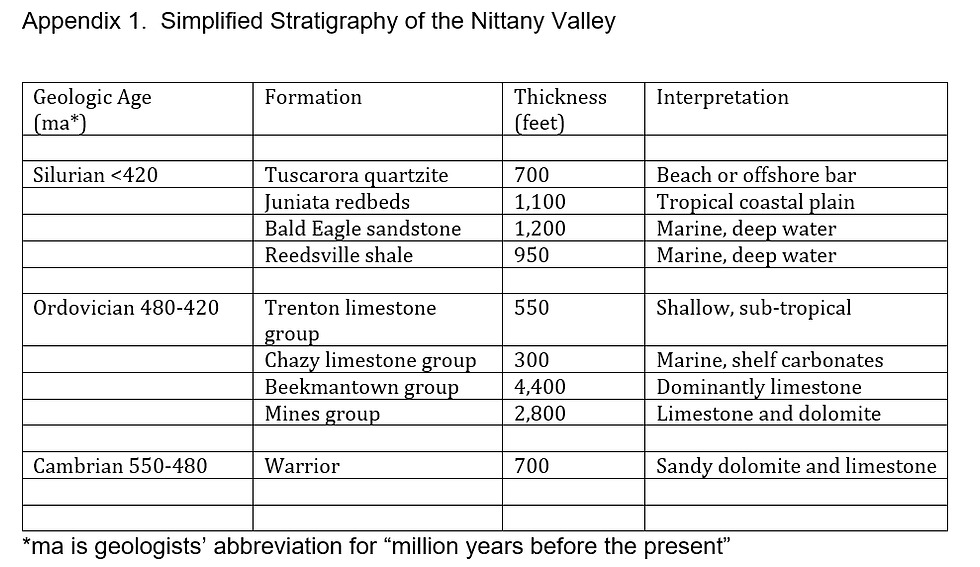
